Thank you for visiting nature.com. You are using a browser version with limited support for CSS. To obtain the best experience, we recommend you use a more up to date browser (or turn off compatibility mode in Internet Explorer). In the meantime, to ensure continued support, we are displaying the site without styles and JavaScript.
Scientific Reports volume 13, Article number: 10130 (2023 ) Cite this article hand therapy table
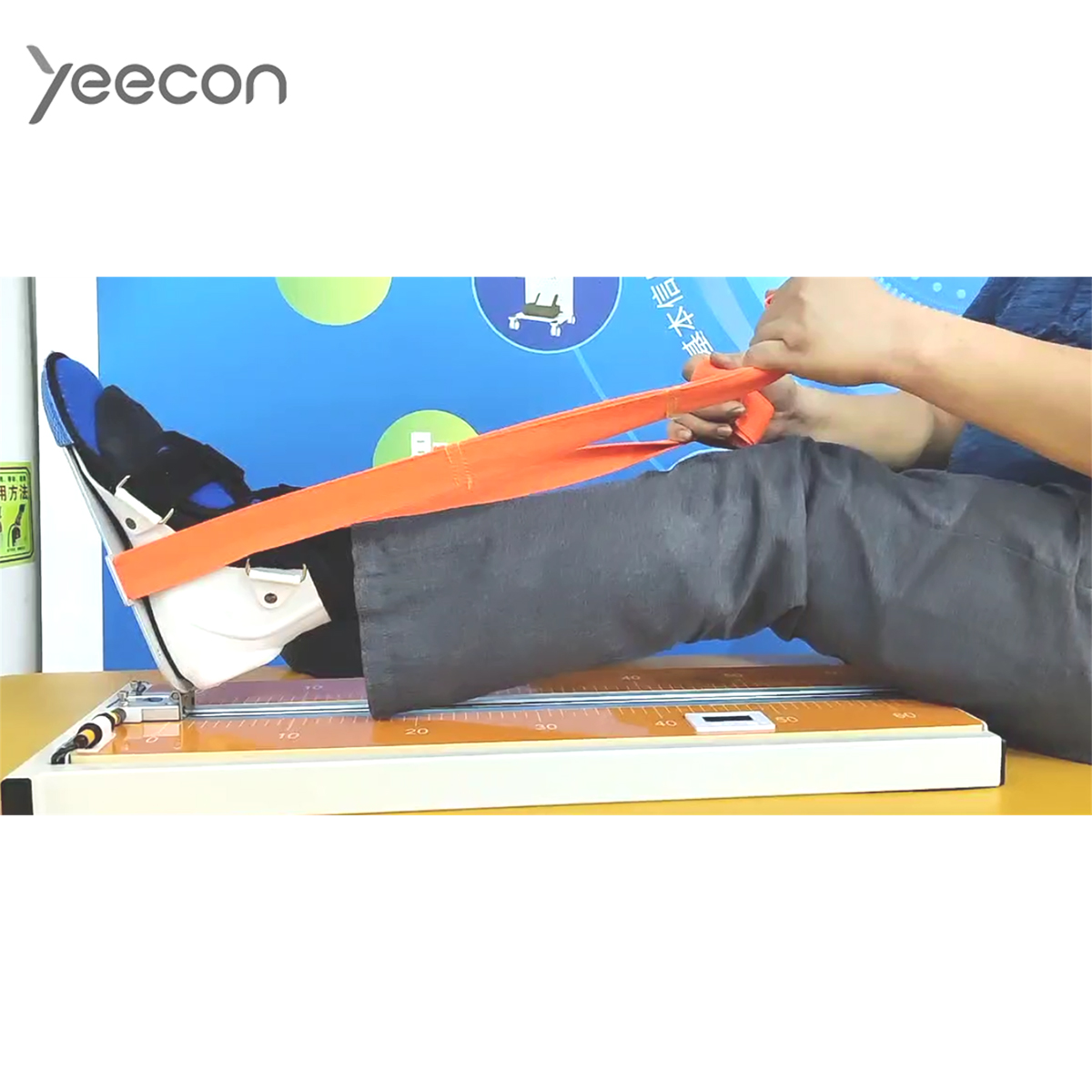
Close links exist between vestibular function and cognition. Dual-task (DT) tests may have ecological validity to assess the impact of daily life cognitive-motor demands in people with vestibular dysfunction (PwVD), functional gait and falls risk. The present paper aimed at building predictive models for functional gait under DT conditions, while clarifying the impact of vestibular dysfunction, individual characteristics, varying task types and motor-cognitive demands. Case-controlled observational study with 39 PwVD and 62 healthy participants. The Functional Gait Assessment (FGA), with and without an additional motor, numeracy, or literacy task, was completed. Multiple linear regression was used to fit models to predict FGA under single and DT performance. Dual task cost (DTC, %) was calculated to assess DT interference on FGA performance using the equation: 100*(single task score–dual task score)/single-task score. Following Bonferroni corrections for multiple comparisons (corrected alpha level of 0.003), PwVD had poorer performance than controls for all FGA conditions (p < 0.001), motor (− 3.94%; p = 0.002) and numeracy (− 22.77%; p = 0.001) DTCs and spatial working memory (p = 0.002). The literacy DTC was marginally significant (− 19.39% p = 0.005). FGA single and DT motor, numeracy, and literacy models explained 76%, 76%, 66% and 67% of the variance respectively for PwVD. Sustained attention, visual memory and sex contributed to all models; short-term visual recognition memory, balance confidence, and migraine contributed to some models. Cognitive performance is impaired in PwVD. Motor, numeracy and literacy tasks impair functional gait performance. Cognitive assessment and FGA with a numeracy or literacy cognitive component should be included within assessment protocols and considered in the provision of targeted interventions for PwVD.
Maya Danneels, Ruth Van Hecke, ... Leen Maes
Taku Matsuura, Kazuhiro Sakashita, … Yasushi Yagi
Your Imaoka, Andrew Flury, ... Eling D. de Bruin
People with vestibular dysfunction (PwVD) often report cognitive symptoms including memory loss, poor concentration, “brain fog” and an inability to multitask1.
Human and animal studies have demonstrated that vestibular disorders are associated with cognitive dysfunction, most prominently in visuospatial and attentional domains but also in executive function, memory, and processing speed1,2,3.
Cognitive rather than mobility tasks more strongly predict activities of daily living impairments in PwVD, and cognitive impairments have a direct impact on activity limitation4. Intriguingly, there is extensive reciprocal connectivity between vestibular and cortical and subcortical areas, raising the possibility of causal links5. However, the exact mechanism that underpins vestibular and cognitive associations remains unclear. Potential mechanisms, not necessarily mutually exclusive, include cortical network atrophy driven by vestibular loss, and involving the hippocampus6,7,8; a top down higher order regulation of vestibular functions by the parieto-temporal and parietal cortex9 that is necessary for spatial representation; the well-established attentional requirements of both static and dynamic balance10; and the presence of bidirectional interactions between psychological state, affective disorders and vestibular symptoms11.
These close interactions are illustrated in the cognitive-motor interference (CMI)12,13 phenomenon, characterized by the deterioration in performance of a cognitive and/or motor task when both are performed simultaneously. CMI can be assessed using a dual-task (DT) testing paradigm7. It is well-established that PwVD require additional attentional resources to maintain balance, thus probably limiting cognitive resources available for other tasks1.
DT studies in PwVD that use “static” sitting or standing balance tasks report varied outcomes ranging from a greater decline in cognitive task performance9 for PwVD versus healthy controls to no difference between groups for either task10; in contrast, the few studies which include a dynamic motor task (i.e. gait) consistently show greater decline in either the motor or cognitive DT for PwVD versus healthy participants8. Dynamic tasks may thus be more sensitive in prompting CMI in PwVD and the complexity of both tasks is crucial14.
Currently CMI in PwVD has only been assessed with DT paradigms incorporating a single, cognitive and motor task. This setting does not represent real-life situations typically involving diverse complex tasks performed during multifaceted gait e.g., talking while avoiding obstacles. Furthermore, no studies in PwVD have considered the effect of confounders such as subjective symptom intensity, cognitive function, and hearing loss on DT performance. In PwVD, lower balance confidence, worse self-perception of handicap and low mood are associated with poorer performance on functional gait measures, deterioration in measurable gait parameters, and/or higher fall incidence15,16,17,18. In healthy adults, worse hearing capacity is associated with poorer performance on the Functional Gait Assessment19, but no studies to date have assessed this in PwVD.
Primary aims of the current study were to extend the DT paradigm to include a standardized complex gait assessment (Functional Gait Assessment, FGA20) in combination with a motor, numeracy and literacy task to a) investigate the effect of various task types and categories on FGA performance and falls risk and b) develop predictive models for single and DT FGA performance in PwVD versus healthy controls. Secondary study aims were to compare cognitive performance between PwVD versus healthy controls on an extended set of visual processing tests, and identify associations between cognitive domains, dynamic gait with and without DTs, and subjective symptoms, psychological state, and demographic variables.
A regional ethical standards committee on human experimentation approved this case-controlled, observational study (Reference: 09/H0718/29) with a priori analysis involving collecting data directly from participants. Strengthening the Reporting of Observational Studies in Epidemiology (STROBE) reporting guidelines were followed21. Written informed consent was obtained from all participants.
Between 2016 and 2019, PwVD were recruited from neuro-otology clinics at the National Hospital for Neurology and Neurosurgery, Queen Square UK, after a complete neurological and neuro-otological examination, including Hallpike positional testing, pure-tone air-conduction and bone conduction threshold audiometry with and without masking, electronystagmography, and caloric testing. In persons with recurrent headaches, migraine was diagnosed according to the International Headache Society International Classification of Headache Disorders 3rd edition diagnostic criteria22; vestibular migraine (VM) was diagnosed if symptoms fit Bárány society criteria23. Inclusion criteria were (1) clinical diagnosis of a peripheral vestibular disorder, (2) chronic dizziness and/or unsteadiness, (3) age 18 to 80 years old, and (4) no completion of a vestibular rehabilitation program. Patients with (1) central nervous system involvement, excluding migraine diagnosed as per the International Headache Society International Classification of Headache Disorders 3rd edition diagnostic criteria22; (2) fluctuating symptoms, for example, active Ménière’s disease; and (3) blindness; (4) severe or profound hearing loss in the better hearing ear (i.e., average of pure tone hearing threshold levels at 250, 500, 1000, 2000 and 4000 Hz that exceeds 71 decibels hearing level) and (5) orthopedic deficit affecting balance and gait were excluded. Patients with severe migraine (> 3 migraine headaches monthly) or severe depression (Hospital Anxiety and Depression (HADS) score ≥ 15/2124) were also excluded.
Independently mobile, healthy participants, aged 18–80 years old, were recruited via posters placed in local community centers and circular university email to staff and students. Exclusion criteria, chosen due to their potential impact on FGA and/or cognitive test performance, included previous diagnosis of a neurological or vestibular disorder, hearing loss, migraine, cognitive impairment and/or orthopedic/musculoskeletal disorder affecting balance and/or gait.
The primary outcome to be predicted was the FGA20 total score which assesses performance on complex gait tasks (i.e. walking with changes in speed, head turns or stepping over obstacles). The FGA total score ranges from 0 to 30 with higher scores indicating better performance. It has shown to be reliable and valid for use in PwVD20. Secondary outcomes to be predicted were the FGA DT conditions. The FGA assessment was completed four times in total for each participant: in isolation (FGA single) and while simultaneously performing a motor (FGA-M), literacy (FGA-L) or numeracy (FGA-N) task. The original FGA was always completed first, followed by the DT FGA in computer generated random order (www.randomizer.org). The FGA-M was performed using the dominant hand to hold a half full cup of water with the elbow flexed at 90°. The cognitive FGA-N DT involved a. counting backwards from 100 in 7’s, b. reciting the 8-multiplication table and c. reciting 7 division tables. The cognitive FGA-L DT involved reciting alternate a. alphabet letters, b. days in a week and c. months in a year. Participants performed the cognitive DTs in the order of a → b → c → a. When repeating the same task, participants started from the number, alphabet, day, or month where they finished the previous time.
Predictor variables were identified by the clinical experts based on expert clinical knowledge25 and choices were supported by scientific evidence which demonstrates an association between the predictor variable with gait performance and/or DT ability. These variables included age, sex, diagnosis, hearing loss, migraine history, cognitive function measures, subjective symptoms, and psychological state15,16,18,19,26,27,28,29. Each participant completed the following tests and self-report measures.
The Cambridge Neuropsychological Test Automated Battery (CANTAB) software (Cambridge Cognition, Cambridge, UK)30, a semiautomated computer program that employs touch screen technology, assessed cognitive function. The cognitive tests performed were rapid visual information processing (RVP); paired associates learning (PAL); spatial working memory (SWM), reaction time (RTI), and delayed matching to sample (DMS). A brief description of each test, what it measures, and data obtained is included in Table 1.
All participants completed validated self-report measures regarding symptoms, symptom triggers, balance confidence and psychological state. The Activities-specific Balance Confidence scale (ABC) assesses balance confidence in 16 daily activities with various difficulty levels31. Scores range from 0 (no confidence) to 100 (complete confidence). The HADS is a 14-item scale consisting of two subscales to assess symptoms of anxiety disorders (HADS-A) and depression (HADS-D)32. Scores between 8-10/21 indicate mild, 10-14/21 moderate and 15-21/21 severe depression or anxiety symptoms, respectively24. The Vertigo Symptom Scale (VSS) assesses frequency and severity of common vestibular (VSS-V; e.g. vertigo, imbalance) and autonomic/somatic (VSS-A; e.g. heart pounding, heavy feeling in the arms or legs) symptoms33. The Situational Characteristics Questionnaire (SCQ) measures symptom provocation or exacerbation frequency in environments with visual-vestibular conflict or intense visual motion (i.e., crowds)34. The Dizziness Handicap Inventory (DHI) is a 25-item scale which quantifies the impact of dizziness on ADLs by evaluating self-perceived handicap in PwVD and includes an emotional (DHI-E), functional (DHI-F) and physical (DHI-P) domain35. Total score ranges from 0 to 100 with higher score indicating greater handicap35. Only the total DHI score was considered as a predictor variable as it is more reliable than scores for individual subscales36,37.
IBM SPSS version 26 (IBM Corp, Armonk, New York, USA) was used for statistical analysis. All data are presented as mean ± SD and median and interquartile range. The data was analyzed using a variety of statistical tests and techniques. First, the chi-square test and Mann–Whitney U test were performed to examine how demographic information differed between the study groups. To test the normality of the distribution for variables, the Shapiro–Wilk test, histogram, and Q-Q plots were used. The results indicated that the data was not normally distributed, therefore, the Mann–Whitney non-parametric test was selected to compare variables between study groups. The effect size was calculated for the Mann–Whitney U test, the standardized test statistic z is divided by the square root of the number of pairs (n). In addition, multiple comparisons can increase the likelihood of Type I errors38, and therefore, it is important to adjust p-values to control for such errors. In this study, 19 Mann–Whitney U tests were conducted on a single group variable. To account for multiple comparisons, a Bonferroni correction was applied. The corrected alpha level was calculated by dividing the desired overall alpha level (0.05) by the number of tests performed (19), resulting in a new corrected alpha level of 0.003.
Within-group DTC differences were assessed using Wilcoxon signed-rank test. Spearman’s correlation assessed for relationships between cognitive performance, self-report measures and demographic variables (age and sex for both groups and migraine history and hearing loss only for PwVD).
The dual task cost (DTC) was calculated to assess DT interference. DTC is the percentage change in FGA performance due to the DT condition and was calculated separately for FGA-M, FGA-N and FGA-L using the following equation39:
In the current study, a more negative DTC indicates a higher impact of adding a secondary task on the primary task (FGA), as a higher FGA score is better. The DTC was only calculated for the primary motor task (FGA), but not for secondary numeracy and literacy task performance as baseline performance on these tasks was not collected.
Predictive models were developed under a multiple linear regression modelling framework as outcomes were continuous. A backwards selection approach was applied to a full model including all potentially relevant predictors that met assumption criteria to derive FGA single and DT performance models. Assumption criteria were (a) independence of residuals (Durbin Watson test values 1.5–2.5/4); (b) linearity, assessed by partial regression plots and a studentized residuals against predicted values plot; (c) homoscedasticity, assessed by visual inspection of a studentized residuals versus unstandardized predicted values plot; (d) no multicollinearity assessed by tolerance values > 0.1 and no correlations between predictors > 0.7; (e) no significant outliers, assessed by checking for studentized deleted residuals > ± 3 SD, leverage values > 0.2, and values for Cook's distance > 1; and (f) assumption of normality was met, assessed by a Q-Q Plot40. If highly correlated predictors were identified, only one was included in the multivariable modelling; outliers were filtered out of the data set and the multiple regression analysis was re-run. Model performance was evaluated by calculating adjusted R2. Significance level was set at 0.05.
Approval was obtained from the ethics committee of the London-Central National Health Service Research Authority (Reference: 09/H0718/29). The procedures used in this study adhere to the tenets of the Declaration of Helsinki.
Informed consent was obtained from all individual participants included in the study.
Thirty-nine PwVD and 62 healthy participants were recruited. Figure 1 summarizes the flow of participants through the study. In PwVD, 48.72% had a migraine history and 33.3% had hearing loss. Demographic data, including vestibular diagnoses, are in Table 2.
Flow diagram of the study regarding numbers examined for eligibility, confirmed eligible, included in the study, and analyzed.
Between-group differences were observed for mean FGA single (U = 158.00, z = − 7.58, p < 0.001, r = 0.74), motor (U = 122.50, z = − 7.66, p < 0.001, r = 0.76), literacy (U = 345.50, z = − 6.04, p < 0.001, r = 0.60) and numeracy (U = 361.00. z = − 5.93, p < 0.001, r = 0.54) scores (Table 3), with poorer performance in PwVD. DTCs were also increased in PwVD relative to controls for FGA motor (U = 767.50. z = − 3.17, p = 0.002, r = 0.32), literacy (U = 807.50, z = − 2.80, p = 0.005, r = 0.28) and numeracy (U = 743.50, z = − 3.25, p = 0.001, r = 0.32) (Table 3). When the Bonferroni correction is applied, the between-group difference for DTC is significant only for FGA motor and FGA numeracy.
Substantial within-group differences were observed for FGA single with FGA-L (Healthy: Z = − 5.99, p < 0.001; PwVD: Z = − 5.12, p < 0.001) and FGA-N (Healthy: Z = − 6.15, p < 0.001; PwVD: Z = − 5.14, p < 0.001) in both groups. Substantial within-group differences for DTC were noted for both groups between FGA-M with FGA-N (Healthy: Z = − 6.36, p < 0.001; PwVD: Z = − 4.62, p < 0.001) and FGA-L (Healthy: Z = − 5.93, p < 0.001; PwVD: Z = − 4.45, p < 0.001). After Bonferroni correction is applied, the within-group differences observed only for PwVD between FGA with FGA-M (Z = − 2.67, p = 0.008;) and between FGA-L and FGA-N (Z = − 2.47, p = 0.013) were no longer significant.
Between-group differences (Table 3) were noted for spatial working memory (U = 783.00, z = − 2.975, p = 0.003, r = 0.28), paired associates learning (U = 897.00, z = − 2.18, p = 0.029, r = 0.22) and reaction time (U = 904.50, z = − 2.12, p = 0.034, r = 0.21). After applying the Bonferroni correction, the between-group difference remains significant only for spatial working memory. No significant between-group differences were noted for delayed matching to (U = 1002.00, z = − 1.45, p = 0.147, r = 0.14) and rapid visual information processing (U = 1025.50, z = − 1.16, p = 0.246, r = 0.12). Between-group differences (p < 0.001) were also observed for all self-report measures (Table 3): balance confidence (ABC, U = 241.50, z = − 6.76, r = 0.67), dizziness handicap total score (DHI total, U = 2.00, z = − 9.06, r = 0.90), vestibular symptoms (VSSV, U = 33.50, z = − 8.43, r = 0.84), autonomic symptoms (VSSA, U = 243.50, z = − 6.65, r = 0.66), visual induced dizziness (SCQ, U = 125.00, z = − 7.59, r = 0.76). anxiety (HADS-A, U = 528.50, z = − 4.76, r = 0.47) and depression (HADS-D, U = 346.00, z = − 6.08, r = 0.60).
Only correlations that remain significant after application of the Bonferroni correction are reported. In healthy participants’, increasing age was associated with poorer performance on mean scores for delayed matching to sample (r = − 0.43, p = 0.001) and rapid visual information processing (r = 0.37, p = 0.003). In PwVD, after applying the Bonferroni corrected alpha level, no significant correlations were identified between cognitive function scores and other variables.
For all FGA models, assumptions of linearity, homoscedasticity, multicollinearity, normality, and independence of residuals (Durbin-Watson: FGA = 1.78, FGA-M = 2.03, FGA-L = 2.05, FGA-N = 2.22) were confirmed. Outliers with studentized deleted residuals > ± 3 SD were identified for FGA single (n = 3), FGA-M (n = 2), FGA-N (n = 2) and FGA-L (n = 4) and were excluded from multiple regression analyses.
Spatial working memory and simple reaction time significantly predicted FGA single, F(2,55) = 9.20, adjusted R2 = 0.22, p < 0.005 and FGA-N, F(2,56) = 10.06, adjusted R2 = 0.24, p < 0.005 (Table 4). Age was the only significant predictor for FGA-M, F(1,57) = 10.11, adjusted R2 = 0.14, p = 0.002 (Table 4). Sex and paired associates learning explained 18% of the variance for FGA-L, F(2,55) = 7.39, adjusted R2 = 0.18, p = 0.001 (Table 4). Variables added significantly to the prediction model for FGA single and all FGA DT conditions (p < 0.05).
For all FGA models, assumptions of linearity, homoscedasticity, normality and independence of residuals (Durbin-Watson: FGA = 2.07, FGA-M = 2.47, FGA-L = 2.08, FGA-N = 1.5) were confirmed. Multicollinearity was violated with correlations > 0.7 between ABC with DHI total score, DHI total score with HADS-D, HADS-A with HADS-D, SCQ with VSSA, VSSV with VSSA resulting in DHI total score, HADS-D and VSSA being excluded from all models. Outliers with studentized deleted residuals > ± 3 SD were identified for FGA single (n = 2), FGA-M (n = 2) and FGA-N (n = 1) and FGA-L (n = 1) and were excluded from the relevant multiple regression analyses.
Rapid visual information processing, paired associates learning, ABC, age, sex and hearing loss significantly predicted FGA single, F(5, 31) = 23.92, p < 0.001, adjusted R2 = 0.76 (Table 5) and FGA-M, F(5,32) = 22.74, adjusted R2 = 0.76, p < 0.001 (Table 5). Rapid visual information processing, paired associates learning, ABC, sex and migraine significantly predicated FGA-L in the initial model, F(5,32) = 16.04, adjusted R2 = 0.67, p < 0.001 while rapid visual information processing, paired associates learning, delayed matching to sample, sex, and migraine significantly predicted FGA-N, F(5,32) = 15.61, adjusted R2 = 0.66, p < 0.001 (Table 5). All variables added significantly to the prediction models for each FGA single and DT condition (p < 0.05).
Regression coefficients and standard errors are in Tables 4 and 5 for healthy participants and PwVD, respectively.
This study investigated the effect of motor and cognitive DTs on complex gait performance, as assessed by FGA, in both PwVD and healthy participants. All PwVD had chronic symptoms and our findings are only applicable to this population. Results showed more pronounced FGA DTCs for cognitive (numeracy and language) versus motor tasks in both groups. Rapid visual processing, paired associates learning, and gender were predictors of FGA performance under both single and DT conditions in PwVD.
Impaired spatial working memory was noted for PwVD compared to healthy participants, which is in agreement with findings reported in previous work in persons with unilateral vestibular loss41. Our finding of impaired paired associates learning in PwVD compared to healthy participants was no longer significant following Bonferroni correction. This may be due to under powering of the study. Worse performance for paired associated learning in PwVD is reported in the animal literature; rats use self-motion (vestibular) signals to disambiguate between spatial locations to form object-place associations42. The pathway involved may include the medial temporal lobe, which is responsible for the visually induced self-perception of motion43 and is activated by sensory conflict between visual and vestibular stimuli44. Interestingly, bilateral vestibular deafferentation leads to changes in the biogenic amine pathways (serotonin/tryptophan) of the medial temporal lobe45. It is an intriguing question whether worse paired associates learning scores in PwVD versus healthy participants may be part of vestibular sensory driven cognitive decline, but both the presence of worse learning scores in PwVD and such a potential association would need to be further investigated. Paired associates learning also predicted all FGA single and DT conditions in PwVD and FGA-L in healthy participants possibly because both gait and an object-space association memory task are dependent on self-motion vestibular (idiothetic) perception42.
Given the predictive role of specific cognitive domains for FGA single and DT scores, an area of potentially fruitful research would be studying whether vestibular rehabilitation has positive cognitive effects. In older adults, physical combined with cognitive training resulted in a greater improvement for paired associates learning versus cognitive training alone46. Vestibular rehabilitation incorporating progressively more challenging functional gait exercises could lead to improved paired associates learning by promoting enhanced perception of self-motion during locomotion and even by possible direct medial temporal lobe activation47. Recently, two exploratory studies assessed vestibular rehabilitation outcomes, in isolation or with the use of a virtual reality head mounted display, in older adults with and without MCI (mild cognitive impairment) and unilateral vestibular hypofunction48,49. The findings suggest that people with MCI benefit from vestibular rehabilitation, with improvements noted in functional gait, postural sway, self-perceived handicap from dizziness and/or quality of life. It appears that vestibular hypofunction is more prevalent in older adults with Alzheimer’s Disease50. Klatt et al.51 state that vestibular rehabilitation might be able to improve balance, and decrease falls, health care costs, and caregiver burden for people with cognitive impairment and have proposed a theoretical and practical guide for vestibular rehabilitation in this population.
Rapid information processing was identified as a predictor for all FGA single and DT models in PwVD while reaction time was not. Reaction time is a simple visual task response latency, while rapid visual information processing is the response speed for detecting a target number sequence. The reaction time task is reliant on dopamine pathways as the dopamine 4 receptor gene and a DRD4 polymorphism is associated with attentional disorders52, while rapid visual information processing is reliant on the cholinergic pathway53.
Current findings for PwVD indicate that dynamic gait +/− dual tasking is more “effortful” for this population1. Paired associates learning and rapid visual information processing which are related to perception and sensory stimuli predicted FGA single and all DT conditions. These changes in cognitive domains may delay PwVD’s ability to encode and embed new information in memory during tasks that require a less practiced task strategy, thus having a negative impact on FGA and DT performance.
In healthy participants, spatial working memory and reaction time predicted FGA single and FGA-N. A small association has previously been observed between gait and spatial working memory54 while processing speed has been shown to contribute to stepping errors55 and stride length56 in older adults. In our study, the predictive role of these cognitive domains is present irrespective of age. However, predictive model effect sizes were weak for healthy participants and results must be considered with caution.
Increasing age is associated with poorer FGA performance in healthy adults28. The weak predictive role for age in healthy participants is likely due to the predominantly younger age of these individuals in the current versus previous studies28,57. Age predicated FGA single and the FGA motor DT condition with older adults having worse scores in PwVD; however, age was not identified as a predictor for cognitive FGA DT conditions. Similar findings have previously been reported whereby the gait DTC increases more in younger versus older adults for a numeracy DT activity58. The authors hypothesised that older adults may have reached their maximal resource capacity with the numeracy task and subsequently showed minimal changes in the gait speed DTC as task difficulty increased from counting backwards in 3’s to 7’s58. It has been shown that compared to younger adults, older persons consume more neural resources to perform simple tasks58 and are therefore likely to achieve the ceiling effect under high demand conditions58,59. We hypothesise that in PwVD the ceiling effect for age was achieved with the FGA in isolation and therefore the further impact of age on numeracy and literacy DT FGA conditions was insignificant.
Sex was a predictive factor for all FGA conditions in PwVD with females having worse FGA single and DT scores. Sex specific gait strategies in response to a physiologic impairment have also been observed. Age-related decreases in saccular function are associated with an increase and decrease in gait speed for men and women, respectively, which may explain the sex impact in all FGA conditions for PwVD60. Recently though significant magnitude and pattern differences in hip, knee and ankle kinematics and kinetics have been reported for healthy women and men, of varying ages, between 20 and 75 years old61. The differences in gait kinetics and kinematics were irrespective of age category and the findings suggest that it is important to consider sex-specific analyses in gait studies62. It may be that kinematic and kinetic sex-specific differences have a distinct impact on functional gait in PwVD and further work is required to assess the impact of sex on functional single and DT gait in this population.
In healthy participants, sex was a predictive factor for FGA-L with women achieving better FGA scores. Sex specific differences have been reported in healthy adults for verbal fluency tasks that require participants to switch categories, with women performing better than men60. The literacy task involved switching between reciting alternate alphabet letters, months or days of the week. Thus, the literacy task may have been easier for healthy women versus men resulting in less DT interference and better FGA scores for the former.
Balance confidence independently predicted FGA performance, except FGA-N in PwVD. Decreased balance confidence in performing functional activities is associated with actual balance performance in older adults with vestibular dysfunction63. Balance confidence contributes to self-efficacy, a person’s belief in their ability to succeed in a particular situation64. Self-efficacy plays an important role in the effort applied to a task and stress experienced when presented with a challenge64. Persons with decreased balance confidence and self-efficacy may modify their behavior to avoid activities and situations that increase symptoms and/or falls risk64. PwVD avoid head movement, physical activity, travel, and social commitments to mitigate symptoms63. Thus, understanding the relationship between balance confidence with FGA and specific cognitive domains, and addressing this together with self-efficacy should be an important interventional target which may result in improved management.
Self-efficacy has been identified as a mediator of the relationship between cognitive ability and conscientiousness with performance65. However, the magnitude of these relationships varies with task complexity. Self-efficacy has been found to mediate the relationships of cognitive ability and conscientiousness with performance on less challenging tasks, but not on more complex tasks65. Chen et al.65 suggest that as tasks become broader and more complex, more generalized, individual, differences influence task performance better than task-specific constructs, such as self-efficacy. This may explain why balance confidence did not predict FGA-N, the most challenging DT condition, in the current study.
Migraine independently predicted FGA-N and FGA-L, with the positive β coefficient for migraine (Table 5) suggesting that it is associated with higher (i.e., better) FGA scores. Although cognitive deficits particularly for memory and attention have frequently been reported during the pre-ictal and ictal migraine phase66,67, findings during the interictal migraine phase have been divisive66,68,69. Factors including migraine frequency, psychological comorbidity, and sample size may account for the heterogeneity of these findings with those experiencing more frequent migraines together with increased psychological symptoms performing worse67. A recent study described better performance for visuospatial memory and learning abilities in persons with migraine compared to healthy controls indicating a “cognitive advantage” in those with migraine who have a low frequency of attacks per month66, as in our study. Better scores for cognitive task performance have been reported in middle age and older adults with migraine for both global measures of cognition and domain specific findings for executive function and susceptibility to interference70. In the present study, none of the persons with migraine history experienced > 3 migraines per month and anxiety and depression symptom scores were within normal levels, which may have contributed to migraine being identified as an independent predictor for FGA-N and FGA-L. However, the sample size for persons with migraine is small and further work is needed to confirm these findings.
Average FGA single and DT scores were significantly worse in PwVD compared to healthy participants in our study and to age-range normative values for the FGA single published previously28. Cut-off scores for falls risk for the FGA single or DT conditions have not been determined in this population. However, a 20% or greater DTC for gait velocity has a destabilizing effect and increases falls risk71. The DTC for FGA-N surpassed this percentage threshold and approached it for FGA-L, suggesting an increased falls risk for these DT conditions compared to FGA single and FGA-M. This finding has potential implications for clinical practice where DT functional gait is often not considered within assessment and intervention programs. Currently, no studies in PwVD have included a pre-post intervention DT gait assessment or investigated the impact of DT training on vestibular rehabilitation outcomes.
DT gait assessment sensitivity depends on the cognitive task used. For both groups, the numeracy and literacy tasks incurred significant DTCs in functional gait compared to the motor task, with the highest sensitivity noted for the numeracy task, as has been reported in persons with MCI72 and healthy older adults73. Literacy (i.e., superior part of Broca's area and premotor cortex) and numeracy task (i.e., temporo-parietal regions) cortical networks are distinct. The numeracy, relative to a literacy task may share more cortical networks with gait, thus producing greater changes in FGA performance73. It has been suggested that the left posterior parietal cortex may be involved in sensorimotor integration processes and gait control in real-world conditions74, while in older adult females, temporal lobe activation, especially the hippocampus, is associated with gait adaptability during unaccustomed treadmill walking75. However, in the current study, although the numeracy task had the highest DTC, no significant differences were noted between FGA-N and FGA-L in the healthy group and the difference between the two was no longer significant in PwVD after Bonferroni correction was applied. The high variability noted for the numeracy and literacy DTCs for both groups, as well as the predominantly younger age for the healthy group, may have contributed to this finding.
Tasks differ in their ability to challenge gait14,58,72. Although, in our study, FGA-N had the highest DTC, a low demand numeracy task (i.e. subtracting from 1) may have shown similar results to FGA single as it is more rhythmic and can cue step pattern72. The motor task had the least impact on FGA performance and only a minimal effect on DTC in both groups. Walking while carrying a cup of water has been conceptualized as a single, complex task with one action goal which is to transport the water without any spills14. Postural control requirements for gait cannot be dissociated from those for holding a cup of water, as control for transporting a hand-held object while walking is contingent upon the inertial forces created by the gait cycle which act on the object14. The cup of water represents an additional postural constraint which increases task complexity but not the number of tasks performed and is therefore insufficient to reveal a DT interference effect14. A suitable motor task would be walking while texting on a mobile phone whereby each task goal is separable and can be distinctly measured14.
Performance decrements during DT gait are also associated with a person’s ability to allocate cognitive resources which depends on cognitive task type and gait task complexity14. Task complexity is determined not only by the task’s difficulty level but also the performer’s expertise and abilities14. Thus, a further factor which may have contributed to outcome for all participants was each person’s experience with performance of the particular tasks, which was not quantified.
The current study provides insights into the effect of varying task types on FGA performance in PwVD. Further work is needed to determine the optimum task type and content for gait assessment in PwVD. Predictive factors show similarities and differences for DT FGA conditions indicating various DT conditions should be assessed to identify the most appropriate tasks. The proposed DT difficulty framework76 for persons with MCI can be implemented for PwVD and used to guide clinicians in choosing appropriate tasks to progressively increase cognitive challenge to identify deficits76. The poorer performance for specific cognitive domains and their impact on single and DT FGA performance, particularly for FGA-N and FGA-L, indicates a need for cognition and functional gait in combination with a cognitive task to be included within a clinician’s assessment in PwVD. In persons who experience dizziness and balance problems following a mild traumatic brain injury, subjective cognitive function scores significantly improve pre-post vestibular rehabilitation, although cognitive symptoms persist77. No studies in PwVD have included cognitive function tests pre-post treatment nor is cognition specifically targeted within published vestibular rehabilitation studies. A cognitive and DT FGA assessment may allow for provision of targeted interventions and improved outcomes in future.
Some study limitations are present. Baseline cognitive data was not collected; therefore, DTC on the cognitive task cannot be determined. As secondary task category and content impacts on outcome, future studies in PwVD should investigate the effect of tasks of varying difficulty within categories including auditory tasks. Passive listening to multi-talker babble noise affects FGA performance in young and particularly older adults and those with decreased hearing capacity19. Poor sleep quality78 and low physical activity levels79 have a detrimental impact on gait; these factors were not included, however, their impact on single and DT FGA should be considered clinically and in future work.
This study provides insights into the effect of chronic vestibular disorders on cognition and DT functional gait. Clinicians should be aware of the additional negative impact of literacy and numeracy tasks on functional gait performance in PwVD. In PwVD, poorer cognitive scores are noted for paired associates learning, reaction time and spatial working memory, irrespective of age, albeit after Bonferroni correction, only the latter remained significant. Gender, varying cognitive domains, balance confidence and migraine history predict FGA single and/or DT performance. The findings support inclusion of a multiple domain cognitive measure and DT FGA that considers various tasks to identify each person’s deficits for the provision of targeted interventions towards optimal management and outcome in PwVD.
Anonymized data will be available by request from qualified researchers whose data use has been approved by an independent review committee. Initial requests should be addressed to Dr Marousa Pavlou at marousa.pavlou@kcl.ac.uk.
Bigelow, R. T. & Agrawal, Y. Vestibular involvement in cognition: Visuospatial ability, attention, executive function, and memory. J Vestib Res. 25(2), 73–89. https://doi.org/10.3233/VES-150544 (2015).
Smith, P. F. & Zheng, Y. From ear to uncertainty: vestibular contributions to cognitive function. Front. Integr. Neurosci. 7, 84. https://doi.org/10.3389/fnint.2013.00084 (2013).
Article PubMed PubMed Central Google Scholar
Semenov , YR , Bigelow , RT , Xue , QL , du Lac , S. & Agrawal , Y. Association between vestibular and cognitive function in US adults: data from the National Health and Nutrition Examination Survey .J. Gerontol.The Biol.Sci.Med.Sci.71(2), 243–250.https://doi.org/10.1093/gerona/glv069 (2016).
Bigelow, R. T., Semenov, Y. R., du Lac, S., Hoffman, H. J. & Agrawal, Y. Vestibular vertigo and comorbid cognitive and psychiatric impairment: the 2008 National Health Interview Survey. J. Neurol. Neurosurg. Psychiatry 87(4), 367–372. https://doi.org/10.1136/jnnp-2015-310319 (2016).
Machado, M. L. et al. Spatial and non-spatial performance in mutant mice devoid of otoliths. Neurosci. Lett. 522(1), 57–61. https://doi.org/10.1016/j.neulet.2012.06.016 (2012).
Article CAS PubMed Google Scholar
Hitier, M., Besnard, S. & Smith, P. F. Vestibular pathways involved in cognition. Front. Integr. Neurosci. 8, 59. https://doi.org/10.3389/fnint.2014.00059 (2014).
Article PubMed PubMed Central Google Scholar
Abernethy, B. Dual-task methodology and motor skills research: some applications and methodological constraints. J. Human Movement Stud. 14, 101–132 (1988).
Nascimbeni , A. , Gaffuri , A. , Penno , A. & Tavoni , M. Dual task interference during gait in patients with unilateral vestibular disorders .J. Neuroeng.Rehabil.7, 47. https://doi.org/10.1186/1743-0003-7-47 (2010).
Article PubMed PubMed Central Google Scholar
Redfern, M. S., Talkowski, M. E., Jennings, J. R. & Furman, J. M. Cognitive influences in postural control of patients with unilateral vestibular loss. Gait Posture. 19(2), 105–114. https://doi.org/10.1016/S0966-6362(03)00032-8 (2004).
Yardley, L. et al. Attentional demands of continuously monitoring orientation using vestibular information. Neuropsychologia 40(4), 373–383 (2002).
Harun , A. , Semenov , YR & Agrawal , Y. Vestibular function and activities of daily living: Analysis of the 1999 to 2004 National Health and Nutrition Examination Surveys.Gerontol.Geriatr.Med.https://doi.org/10.1177/2333721415607124 (2015).
Article PubMed PubMed Central Google Scholar
Andersson, G., Hagman, J., Talianzadeh, R., Svedberg, A. & Larsen, H. C. Dual-task study of cognitive and postural interference in patients with vestibular disorders. Otol. Neurotol. 24(2), 289–293. https://doi.org/10.1097/00129492-200303000-00026 (2003).
Bessot, N., Denise, P., Toupet, M., Van Nechel, C. & Chavoix, C. Interference between walking and a cognitive task is increased in patients with bilateral vestibular loss. Gait Posture 36(2), 319–321. https://doi.org/10.1016/j.gaitpost.2012.02.021 (2012).
McIsaac, T. L., Lamberg, E. M. & Muratori, L. M. Building a framework for a dual task taxonomy. Biomed. Res. Int. 2015, 591475. https://doi.org/10.1155/2015/591475 (2015).
Article PubMed PubMed Central Google Scholar
Zanotto, D. et al. Dizziness handicap inventory score is highly correlated with markers of gait disturbance. Otol Neurotol. 38(10), 1490–1499. https://doi.org/10.1097/MAO.0000000000001586 (2017).
Herssens, N. et al. The relationship between the activities-specific balance confidence scale and balance performance, self-perceived handicap, and fall status in patients with peripheral Dizziness or imbalance. Otol. Neurotol. 42(7), 1058–1066. https://doi.org/10.1097/MAO.0000000000003166 (2021).
Vereeck, L., Truijen, S., Wuyts, F. L. & Van de Heyning, P. H. The dizziness handicap inventory and its relationship with functional balance performance. Otol. Neurotol. 28(1), 87–93. https://doi.org/10.1097/01.mao.0000247821.98398.0d (2007).
MacDowell, S. G. et al. The impact of symptoms of anxiety and depression on subjective and objective outcome measures in individuals with vestibular disorders. J. Vestib. Res. 27(5–6), 295–303. https://doi.org/10.3233/VES-170627 (2018).
Buyle, M., Azoidou, V., Pavlou, M., Van Rompaey, V. & Bamiou, D. E. Functional gait can be affected by noise: Effects of age and cognitive function: a pilot study. Front Neurol. 12, 634395. https://doi.org/10.3389/fneur.2021.634395 (2021).
Article PubMed PubMed Central Google Scholar
Wrisley, D. M., Marchetti, G. F., Kuharsky, D. K. & Whitney, S. L. Reliability, internal consistency, and validity of data obtained with the functional gait assessment. Phys Ther. 84(10), 906–918 (2004).
von Elm, E. et al. The Strengthening the Reporting of Observational Studies in Epidemiology (STROBE) statement: guidelines for reporting observational studies. Rev. Esp. Salud Publica 82(3), 251–259. https://doi.org/10.1590/s1135-57272008000300002 (2008).
Headache Classification Committee of the International Headache Society (IHS) The International Classification of Headache Disorders, 3rd edition. Cephalalgia 38(1), 1–211 (2018). https://doi.org/10.1177/0333102417738202
Lempert, T. et al.Vestibular migraine: diagnostic criteria.J. Vestib.Res. 22(4), 167–172.https://doi.org/10.3233/VES-2012-0453 (2012).
Bjelland, I., Dahl, A. A., Haug, T. T. & Neckelmann, D. The validity of the Hospital Anxiety and Depression Scale. An updated literature review. J. Psychosom. Res. 52(2), 69–77. https://doi.org/10.1016/s0022-3999(01)00296-3 (2002).
Moons, K. G. et al. Risk prediction models: I. Development, internal validation, and assessing the incremental value of a new (bio)marker. Heart 98(9), 683–690. https://doi.org/10.1136/heartjnl-2011-301246 (2012).
Baker , BJ , Curtis , A. , Trueblood , P. & Vangsnes , E. Vestibular functioning and migraine: Comparing those with and without vertigo to a normal population .J. Laryngo.Otol.127(12), 1169–1176.https://doi.org/10.1017/S0022215113002302 (2013).
Article CAS PubMed Google Scholar
Verghese, J. et al. Gait dysfunction in mild cognitive impairment syndromes. J. Am. Geriatr. Soc. 56(7), 1244–1251. https://doi.org/10.1111/j.1532-5415.2008.01758.x (2008).
Article PubMed PubMed Central Google Scholar
Walker, M. L. et al. Reference group data for the functional gait assessment. Phys. Ther. 87(11), 1468–1477. https://doi.org/10.2522/ptj.20060344 (2007).
Agmon, M., Armon, G., Denesh, S. & Doumas, M. The role of gender in the association between personality and task priority in older adults’ dual-tasking while walking. BMC Geriatr. 18(1), 1. https://doi.org/10.1186/s12877-017-0691-1 (2018).
Article PubMed PubMed Central Google Scholar
Sahakian, B. J. & Owen, A. M. Computerized assessment in neuropsychiatry using CANTAB: discussion paper. J. R. Soc. Med. 85(7), 399–402 (1992).
CAS PubMed PubMed Central Google Scholar
Powell, L. E. & Myers, A. M. The activities-specific balance confidence (ABC) scale. J. Gerontol. A Biol. Sci. Med. Sci. 50A(1), M28-34 (1995).
Article CAS PubMed Google Scholar
Zigmond, A. S. & Snaith, R. P. The hospital anxiety and depression scale. Acta Psychiatr. Scand. 67(6), 361–370 (1983).
Article CAS PubMed Google Scholar
Yardley, L., Masson, E., Verschuur, C., Haacke, N. & Luxon, L. Symptoms, anxiety and handicap in dizzy patients: development of the vertigo symptom scale. J. Psychosom. Res. 36(8), 731–741 (1992).
Article CAS PubMed Google Scholar
Guerraz, M. et al. Visual vertigo: symptom assessment, spatial orientation and postural control. Brain 124(Pt 8), 1646–1656 (2001).
Article CAS PubMed Google Scholar
Jacobson, G. P. & Newman, C. W. The development of the Dizziness Handicap Inventory. Arch. Otolaryngol. Head Neck Surg. 116(4), 424–427 (1990).
Article CAS PubMed Google Scholar
Kurre, A. et al. Exploratory factor analysis of the Dizziness Handicap Inventory (German version). BMC Ear Nose Throat Disord. 10, 3. https://doi.org/10.1186/1472-6815-10-3 (2010).
Article PubMed PubMed Central Google Scholar
Kammerlind, A. S. L. P., Ledin, T. & Skargren, E. Reliability of clinical balance tests and subjective ratings in dizziness and disequilibrium. Adv. Physioth. 7, 96–107 (2005).
Rothman, K. J. No adjustments are needed for multiple comparisons. Epidemiology 1(1), 43–46 (1990).
Article CAS PubMed Google Scholar
Plummer-D’Amato, P. & Altmann, L. J. Relationships between motor function and gait-related dual-task interference after stroke: a pilot study. Gait Posture 35(1), 170–172. https://doi.org/10.1016/j.gaitpost.2011.08.015 (2012).
Field, A. P. Discovering Statistics Using SPSS: and Sex and Drugs and Rock “n” Roll (Sage, 2013).
Popp, P. et al. Cognitive deficits in patients with a chronic vestibular failure. J. Neurol. 264(3), 554–563. https://doi.org/10.1007/s00415-016-8386-7 (2017).
Sanchez, L. M., Thompson, S. M. & Clark, B. J. Influence of proximal, distal, and vestibular frames of reference in object-place paired associate learning in the rat. PLoS ONE 11(9), e0163102. https://doi.org/10.1371/journal.pone.0163102 (2016).
Article CAS PubMed PubMed Central Google Scholar
Becker-Bense, S. et al. Ventral and dorsal streams processing visual motion perception (FDG-PET study). BMC Neurosci. 13, 81. https://doi.org/10.1186/1471-2202-13-81 (2012).
Article CAS PubMed PubMed Central Google Scholar
Nguyen, N. T. et al. Cerebral hemodynamic responses to the sensory conflict between visual and rotary vestibular stimuli: an analysis with a multichannel near-infrared spectroscopy (NIRS) system. Front. Hum. Neurosci. 14, 125. https://doi.org/10.3389/fnhum.2020.00125 (2020).
Article CAS PubMed PubMed Central Google Scholar
Goddard, M., Zheng, Y., Darlington, C. L. & Smith, P. F. Monoamine transporter and enzyme expression in the medial temporal lobe and frontal cortex following chronic bilateral vestibular loss. Neurosci. Lett. 437(2), 107–110. https://doi.org/10.1016/j.neulet.2008.03.073 (2008).
Article CAS PubMed Google Scholar
Theill, N., Schumacher, V., Adelsberger, R., Martin, M. & Jäncke, L. Effects of simultaneously performed cognitive and physical training in older adults. BMC Neurosci. 14, 103. https://doi.org/10.1186/1471-2202-14-103 (2013).
Article PubMed PubMed Central Google Scholar
Yamamoto, N. et al. Medial temporal lobe roles in human path integration. PLoS ONE 9(5), e96583. https://doi.org/10.1371/journal.pone.0096583 (2014).
Article ADS CAS PubMed PubMed Central Google Scholar
Micarelli, A. et al. Gradient impact of cognitive decline in unilateral vestibular hypofunction after rehabilitation: preliminary findings. Eur. Arch. Otorhinolaryngol. 275(10), 2457–2465. https://doi.org/10.1007/s00405-018-5109-y (2018).
Micarelli, A., Viziano, A., Micarelli, B., Augimeri, I. & Alessandrini, M. Vestibular rehabilitation in older adults with and without mild cognitive impairment: Effects of virtual reality using a head-mounted display. Arch. Gerontol. Geriatr. 83, 246–256. https://doi.org/10.1016/j.archger.2019.05.008 (2019).
Harun , A. , Oh , ES , Bigelow , RT , Studenski , S. & Agrawal , Y. Vestibular impairment in dementia .Neurotol Otol.37(8), 1137–1142.https://doi.org/10.1097/MAO.0000000000001157 (2016).
Article PubMed PubMed Central Google Scholar
Klatt, B. N., Ries, J. D., Dunlap, P. M., Whitney, S. L. & Agrawal, Y. Vestibular physical therapy in individuals with cognitive impairment: a theoretical framework. J. Neurol. Phys. Ther. 43(Suppl 2), S14–S19. https://doi.org/10.1097/NPT.0000000000000266 (2019).
Article PubMed PubMed Central Google Scholar
Szekely, A. et al. Genetic factors of reaction time performance: DRD4 7-repeat allele associated with slower responses. Genes Brain Behav. 10(2), 129–136. https://doi.org/10.1111/j.1601-183X.2010.00645.x (2011).
Article CAS PubMed Google Scholar
Wesnes, K. & Warburton, D. M. Effects of scopolamine and nicotine on human rapid information processing performance. Psychopharmacology 82(3), 147–150. https://doi.org/10.1007/BF00427761 (1984).
Article CAS PubMed Google Scholar
Giannouli, E., Bock, O. & Zijlstra, W. Cognitive functioning is more closely related to real-life mobility than to laboratory-based mobility parameters. Eur. J. Ageing 15(1), 57–65. https://doi.org/10.1007/s10433-017-0434-3 (2018).
Caetano, M. J. D. et al. Sensorimotor and cognitive predictors of impaired gait adaptability in older people. J. Gerontol. A Biol. Sci. Med. Sci. 72(9), 1257–1263. https://doi.org/10.1093/gerona/glw171 (2017).
Valkanova, V. et al. Association between gait and cognition in an elderly population based sample. Gait Posture. 09(65), 240–245. https://doi.org/10.1016/j.gaitpost.2018.07.178 (2018).
Wrisley, D. M. & Kumar, N. A. Functional gait assessment: concurrent, discriminative, and predictive validity in community-dwelling older adults. Phys Ther. 90(5), 761–773. https://doi.org/10.2522/ptj.20090069 (2010).
Goh, H. T., Pearce, M. & Vas, A. Task matters: an investigation on the effect of different secondary tasks on dual-task gait in older adults. BMC Geriatr. 21(1), 510. https://doi.org/10.1186/s12877-021-02464-8 (2021).
Article PubMed PubMed Central Google Scholar
Nóbrega-Sousa, P. et al. Prefrontal cortex activity during walking: effects of aging and associations with gait and executive function. Neurorehabil. Neural Repair. 34(10), 915–924. https://doi.org/10.1177/1545968320953824 (2020).
Layman, A. J. et al. Association between saccular function and gait speed: data from the Baltimore Longitudinal Study of Aging. Otol Neurotol. 36(2), 260–266. https://doi.org/10.1097/MAO.0000000000000544 (2015).
Article PubMed PubMed Central Google Scholar
Rowe, E., Beauchamp, M. K. & Astephen, W. J. Age and sex differences in normative gait patterns. Gait Posture. 88, 109–115. https://doi.org/10.1016/j.gaitpost.2021.05.014 (2021).
Uzor, S., Baillie, L., Skelton, D. A. & Rowe, P. J. Falls prevention advice and visual feedback to those at risk of falling: Study protocol for a pilot randomized controlled trial. Trials 14, 79. https://doi.org/10.1186/1745-6215-14-79 (2013).
Article PubMed PubMed Central Google Scholar
Marchetti, G. F., Whitney, S. L., Redfern, M. S. & Furman, J. M. Factors associated with balance confidence in older adults with health conditions affecting the balance and vestibular system. Arch. Phys. Med. Rehabil. 92(11), 1884–1891. https://doi.org/10.1016/j.apmr.2011.06.015 (2011).
Article PubMed PubMed Central Google Scholar
Landers, M. R., Oscar, S., Sasaoka, J. & Vaughn, K. Balance confidence and fear of falling avoidance behavior are most predictive of falling in older adults: Prospective analysis. Phys Ther. 96(4), 433–442. https://doi.org/10.2522/ptj.20150184 (2016).
Chen, G., Casper, W. J. & Cortina, J. The roles of self-efficacy and task complexity in the relationships among cognitive ability, conscientiousness, and work-related performance: a meta-analytic examination. Human Perform. 14(3), 209–230 (2001).
Baschi, R. et al. Visuospatial learning is fostered in migraine: Evidence by a neuropsychological study. Neurol Sci. 40(11), 2343–2348. https://doi.org/10.1007/s10072-019-03973-6 (2019).
Vuralli , D. , Ayata , C. & Bolay , H. Cognitive dysfunction and migraine .J. Headache Pain 19(1), 109. https://doi.org/10.1186/s10194-018-0933-4 (2018).
Article PubMed PubMed Central Google Scholar
Le Pira, F. et al. Memory disturbances in migraine with and without aura: A strategy problem?. Cephalalgia 20(5), 475–478. https://doi.org/10.1046/j.1468-2982.2000.00074.x (2000).
Camarda, C., Monastero, R., Pipia, C., Recca, D. & Camarda, R. Interictal executive dysfunction in migraineurs without aura: Relationship with duration and intensity of attacks. Cephalalgia 27(10), 1094–1100. https://doi.org/10.1111/j.1468-2982.2007.01394.x (2007).
Article CAS PubMed Google Scholar
Wen, K., Nguyen, N. T., Hofman, A., Ikram, M. A. & Franco, O. H. Migraine is associated with better cognition in the middle-aged and elderly: The Rotterdam Study. Eur. J. Neurol. 23(10), 1510–1516. https://doi.org/10.1111/ene.13066 (2016).
Article CAS PubMed Google Scholar
Hollman, J. H., Kovash, F. M., Kubik, J. J. & Linbo, R. A. Age-related differences in spatiotemporal markers of gait stability during dual task walking. Gait Posture 26(1), 113–119. https://doi.org/10.1016/j.gaitpost.2006.08.005 (2007).
Bahureksa, L. et al. The impact of mild cognitive impairment on gait and balance: A systematic review and meta-analysis of studies using instrumented assessment. Gerontology 63(1), 67–83. https://doi.org/10.1159/000445831 (2017).
Li, C., Verghese, J. & Holtzer, R. A comparison of two walking while talking paradigms in aging. Gait Posture 40(3), 415–419. https://doi.org/10.1016/j.gaitpost.2014.05.062 (2014).
Article PubMed PubMed Central Google Scholar
Pizzamiglio, S., Abdalla, H., Naeem, U. & Turner, D. L. Neural predictors of gait stability when walking freely in the real-world. J. Neuroeng. Rehabil. 15(1), 11. https://doi.org/10.1186/s12984-018-0357-z (2018).
Article PubMed PubMed Central Google Scholar
Rosano, C. et al. Patterns of focal gray matter atrophy are associated with bradykinesia and gait disturbances in older adults. J. Gerontol. A Biol. Sci. Med. Sci. 67(9), 957–962. https://doi.org/10.1093/gerona/glr262 (2012).
Article PubMed PubMed Central Google Scholar
Difede, J. et al. Virtual reality exposure therapy for the treatment of posttraumatic stress disorder following September 11, 2001. J. Clin. Psychiatry. 68(11), 1639–1647 (2007).
Søberg, H. L. et al. Effect of vestibular rehabilitation on change in health-related quality of life in patients with dizziness and balance problems after traumatic brain injury: A randomized controlled trial. J. Rehabil. Med. 53(4), jrm00181. https://doi.org/10.2340/16501977-2823 (2021).
Furtado, F., Gonçalves, B. D., Abranches, I. L., Abrantes, A. F. & Forner-Cordero, A. Chronic low quality sleep impairs postural control in healthy adults. PLoS ONE 11(10), e0163310. https://doi.org/10.1371/journal.pone.0163310 (2016).
Article CAS PubMed PubMed Central Google Scholar
Rasmussen, L. J. H. et al. Association of neurocognitive and physical function with gait speed in midlife. JAMA Netw Open 2(10), e1913123. https://doi.org/10.1001/jamanetworkopen.2019.13123 (2019).
Article PubMed PubMed Central Google Scholar
Professor Doris-Eva Bamiou and Dr Marousa Pavlou received funding from the HOLOBALANCE EU Horizon 2020 project (Grant Number 769574). We would like to thank Dr Yanzhong Wang, Reader in Medical Statistics, King’s College London for statistical advice. An independent statistician also reviewed all results.
Professor Doris-Eva Bamiou and Dr Marousa Pavlou received funding from the HOLOBALANCE EU Horizon 2020 project (Grant Number 769574).
Centre for Human and Applied Physiological Sciences, School of Basic and Medical Biosciences, Faculty of Life Sciences and Medicine, King’s College London, Shepherd’s House, Guy’s Campus, London, SE1 1UL, UK
Marousa Pavlou & William Galsworthy
Department of Neuro-Otology, University College London Hospitals, London, UK
Marousa Pavlou, George Korres & Doris-Eva Bamiou
Ear Institute, University College London, London, UK
Biomedical Research Centre, University College London Hospitals, London, UK
Sergi G. Costafreda & Doris-Eva Bamiou
You can also search for this author in PubMed Google Scholar
You can also search for this author in PubMed Google Scholar
You can also search for this author in PubMed Google Scholar
You can also search for this author in PubMed Google Scholar
You can also search for this author in PubMed Google Scholar
Dr M.P. and Professor Doris-Eva Bamiou were responsible for the study conception and design. Material preparation, data collection and analysis were performed by all authors (Dr M.P., Professor D.-E.B., Dr S.C., Dr G.K., Mr W.G.). The first draft of the manuscript was written by Dr M.P. and Dr W.G. and all authors commented on previous versions of the manuscript. All authors read and approved the final manuscript.
The authors declare no competing interests.
Springer Nature remains neutral with regard to jurisdictional claims in published maps and institutional affiliations.
Open Access This article is licensed under a Creative Commons Attribution 4.0 International License, which permits use, sharing, adaptation, distribution and reproduction in any medium or format, as long as you give appropriate credit to the original author(s) and the source, provide a link to the Creative Commons licence, and indicate if changes were made. The images or other third party material in this article are included in the article's Creative Commons licence, unless indicated otherwise in a credit line to the material. If material is not included in the article's Creative Commons licence and your intended use is not permitted by statutory regulation or exceeds the permitted use, you will need to obtain permission directly from the copyright holder. To view a copy of this licence, visit http://creativecommons.org/licenses/by/4.0/.
Pavlou, M., Costafreda, S.G., Galsworthy, W. et al. The interplay between cognition, functional and dual-task gait in persons with a vestibular disorder versus healthy controls. Sci Rep 13, 10130 (2023). https://doi.org/10.1038/s41598-023-35904-z
DOI: https://doi.org/10.1038/s41598-023-35904-z
Anyone you share the following link with will be able to read this content:
Sorry, a shareable link is not currently available for this article.
Provided by the Springer Nature SharedIt content-sharing initiative
By submitting a comment you agree to abide by our Terms and Community Guidelines. If you find something abusive or that does not comply with our terms or guidelines please flag it as inappropriate.
Scientific Reports (Sci Rep) ISSN 2045-2322 (online)
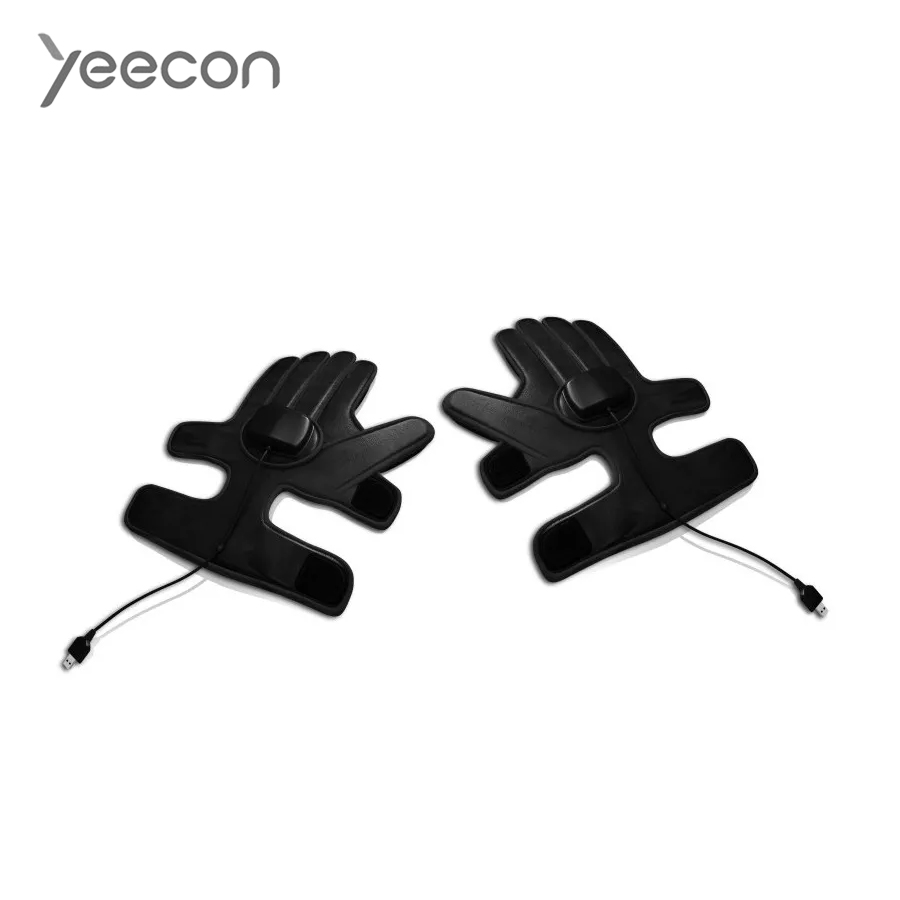
Gait Training Device Sign up for the Nature Briefing newsletter — what matters in science, free to your inbox daily.